
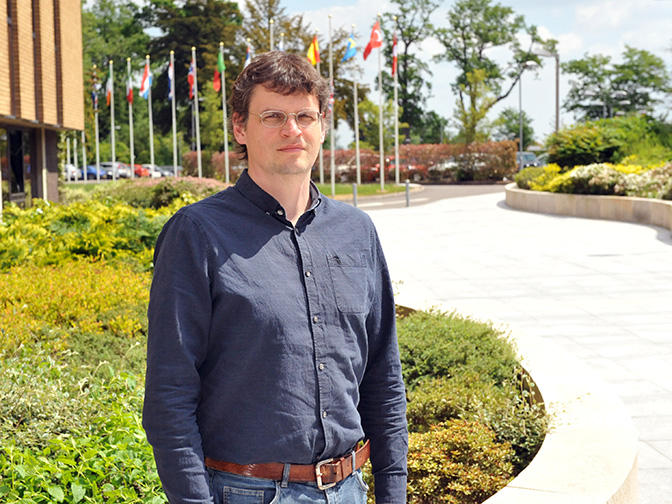
On 17 May 2007, ECMWF scientist Johannes Flemming initiated the Centre’s first atmospheric composition forecast.
Ten years later the CAMS team continues to break new ground to keep improving what has become a successful operational service.
Today the representation of atmospheric composition processes in ECMWF’s Integrated Forecasting System is an important aspect of the Centre’s Earth system approach designed to unlock new sources of predictability and harness a wider range of observations.
Dr Flemming studied meteorology at the Free University of Berlin with physical chemistry as a subsidiary subject.
“I was interested in physics but felt that meteorology was more directly related to the environment in which we live,” he says.
Having obtained a PhD in the use of observations for air pollution forecasts in Germany and Europe, in 2005 he joined an international atmospheric composition project coordinated by ECMWF.
The project for Global and Regional Earth-System Monitoring using Satellite and In-Situ Data (GEMS) aimed to develop forecasting capabilities for aerosols, greenhouse gases and reactive gases.
GEMS was followed by a series of successor projects (MACC I–III) which paved the way for the EU-funded Copernicus Atmosphere Monitoring Service (CAMS) operated by ECMWF.
Today Dr Flemming works as an atmospheric composition scientist for CAMS.
“If you want to predict atmospheric composition, you need to know how different components of the atmosphere interact with each other, with elements of the weather, and with the surface,” he says.
But any model also needs some data input if it is to make realistic predictions.
In the case of atmospheric composition, this comes from emission datasets, numerical weather predictions, and observations of atmospheric composition.
Successful forecasts of atmospheric composition require high-quality input from emission datasets and observations as well as a realistic model of all relevant processes. The chart shown here is a four-day forecast of nitrogen dioxide at the surface in parts per billion by volume (ppbv) for 3 June 2017 00 UTC. It highlights the impact of maritime transport as well as of land-based emissions in different parts of the world.
First composition forecasts
In May 2007, Dr Flemming proposed running routine daily atmospheric composition forecasts at the Centre. This was ahead of schedule compared to the work plan of the GEMS project.
“From my work with regional air pollution forecasting in Berlin I knew that, in atmospheric composition, it is possible to achieve sensible results just on the basis of emission data combined with a chemical transport and weather prediction model,” he says.
His proposal was met with a degree of scepticism because the data assimilation system wasn’t ready yet. Colleagues feared that, without assimilating observations of atmospheric composition to continually adjust the initial conditions, forecasts might be meaningless, as they would have been in the case of weather prediction.
“In the event, the forecast picked up a textbook case of long-range transport across the Atlantic. It became clear from the start that the GEMS system could provide useful information. It was subsequently further improved by assimilating atmospheric composition observations from satellites,” Dr Flemming adds.
Today ECMWF’s data assimilation system plays a vital role in the production of global CAMS forecasts of atmospheric composition.
Every year many millions of tonnes of aerosols and gases are emitted into the atmosphere as a result of human activities in Europe alone. Emission data are fed into atmospheric composition models as boundary conditions. (Photo: Digital Vision/Photodisc/Thinkstock)
Modelling
Model development is another area in which decisive progress has been made since the early days of GEMS.
An important step was to move from a coupled system, in which a chemical transport model was run in parallel with ECMWF’s Integrated Forecasting System (IFS), to a fully integrated one.
This was done first for the troposphere and then also for the stratosphere.
“Today everything happens in the IFS,” Dr Flemming says. “For historical reasons aerosols and gas phase species also used to be modelled separately – but since they interact in nature they are now increasingly treated in an integrated way.”
All development work for the IFS chemistry and aerosol schemes is carried out jointly with partners in Europe, for example from the Dutch national meteorological service (KNMI); the Max Planck Institute for Meteorology in Germany; the French national meteorological service, Météo-France; and the National Center for Scientific Research (CNRS) in France.
“I enjoy this collaborative way of working. Often my role is to evaluate and bring together different development efforts for our Integrated Forecasting System.”
Another dramatic change is an increase in horizontal resolution from 125 km in the early days to 40 km for forecasts and 80 km for reanalysis today.
In June 2016, the horizontal resolution of the global forecasting model used in CAMS was increased from 80 km to 40 km. The charts show 21-hour forecasts of dust aerosol optical depth at 550 nm for 21 UTC on 5 April 2016 at the previous resolution (left) and at the new resolution (right).
Running the model at these resolutions requires significant computing power.
“Fifty-plus chemical species and their interactions have to be modelled and evolved in time, plus 11 aerosols. With chemistry the forecasting part of the IFS requires about fives time more computational resources than without, while for data assimilation the factor is two.”
Emissions, deposition and weather
Emissions of gases and aerosols come from a wide range of sources: they are linked to human activities, such as burning fossil fuels, igniting forest fires and keeping livestock, and to natural processes, such as emissions from plants and volcanic outgassing.
Datasets of such emissions are fed into atmospheric composition models. Uncertainty in their accuracy makes a significant contribution to the uncertainty in atmospheric composition forecasts.
Weather events such as lightning can influence the composition of the atmosphere. (Photo: disqis/iStock/Thinkstock)
Knowing the rate at which substances are emitted into the atmosphere is one issue. Working out at what rate they are removed again is the modeller’s task.
“There are for example wet deposition processes resulting from the presence of clouds and rain, and dry deposition processes resulting from interactions with the surface,” Dr Flemming explains.
“We need to improve the modelling of these processes to reduce biases in the forecasts and also to provide deposition fluxes, which is potentially important information for a range of CAMS users.”
Chemical reactions in the atmosphere may also be facilitated by weather events. For example, the intense heat generated by lightning causes the production of nitrogen monoxide.
“One of the tasks on my to-do list is to test the impact of a new lightning scheme in the IFS on our composition forecasts.”
Assimilating observations
Assimilating satellite observations of atmospheric composition is a key challenge in CAMS.
“My role is to prepare a model which has low biases to ensure the successful assimilation of satellite retrievals.
“The challenge is then to extract the often limited information content of the retrievals to further improve the model’s output. For reactive gases this is the task of my long-time colleague Antje Inness.”
Observations of atmospheric composition can come from satellites or ground-based observation networks.
At ECMWF, satellite observations are used in data assimilation while in situ observations are mainly used to evaluate forecast quality.
“Data assimilation makes a significant difference, especially for substances that stay in the atmosphere for longer than a couple of days,” Dr Flemming emphasises.
It can also be essential to capture sudden changes in composition that are not accounted for by long-term emission datasets.
Volcanic eruptions are an example.
They may spew millions of tonnes of ash and sulphur dioxide into the atmosphere in just a few days.
When the Eyjafjallajökull volcano in Iceland erupted in April in 2010, the resulting plume of emissions spread over thousands of kilometres and brought much of European air traffic to a standstill. The eruption of Iceland’s Grímsvötn volcano a year later also caused significant disruption to air travel. (Photo: sumos/iStock/Thinkstock)
“When Eyjafjallajökull erupted in 2010, Dr Inness and I started to work on predicting the resulting sulphur dioxide plumes by using observations as well as the model,” Dr Flemming says.
“As a result of this work, we now have volcano plume modelling capabilities in research mode. It started as a bit of a hobby-horse of mine. It’s good to have hobbies, even at work!”